Authors: Abraham Kabutey, David Herak, and Cestmir Mizera
Department of Mechanical Engineering, Faculty of Engineering, Czech University of Life Sciences Prague, 165 20 Prague, Czech Republic * Correspondence: kabutey@tf.czu.cz; Tel.: +420-22438-3180
Abstract: The present study aims to estimate the maximum oil yield of hulled sunflower seed samples in a uniaxial process under a load of 40 kN and speed of 4 mm/min. The oil samples were assessed for their quality parameters and spectra curves within the wavelength range of 325–600 nm. The results show that heating temperatures in the range of 40 ◦C to 80 ◦C increased the oil output; however, a maximum oil yield of 48.869 ± 6.023% with a minimum energy of 533.709 ± 65.644 J at the fifth repeated pressing was obtained from the unheated sample compared to the heated samples. The peroxide values ranged from 6.898 ± 0.144 to 7.290 ± 0.507 meq O2/kg, acid values from 1.043 ± 0.166 to 1.998 ± 0.276 mg KOH/g oil and free fatty acid values from 0.521 ± 0.083 to 0.999 ± 0.138 mg KOH/g oil, which were within the recommended quality threshold. There were significant spectral differences among the oil samples. A single absorbance peak was observed at 350 nm for all oil samples, indicating low levels of pigment molecules in the oil. The study revealed the need for repeated pressings to recover the considerable residual oil remaining in the seedcake after the first pressing.
Keywords: edible oilseeds; oil recovery; heating temperatures; laboratory production; quality usage
1. Introduction Edible/vegetable oil consumption is mainly based on palm, soybean, rapeseed and sunflower oil [1,2]. In 2020/2021, palm and soybean were the most utilized vegetable fats worldwide with 38.4% and 34.6% of the vegetable oil market with a consumption rate of 75.45 and 59.48 million metric tons, respectively. In addition, sunflower and rape oils were exploited at 9.7% and 6.3% with a consumption rate of 19.02 and 27.64 million metric tons, respectively [3–5]. According to Ng et al. [6] and Rifna et al. [7], worldwide vegetable oil consumption is projected to be above 170 million tons annually. Globally, sunflower is the third most important oilseed crop after soybean and rapeseed. The crop produces oil for food for human consumption and meal for animal feed as well as for industrial applications such as biodiesel and lubricants [5,8,9]. Vegetable oil can be used as a therapeutic for Alzheimer’s disease [10,11] and prevention of ultraviolet radiation on the human skin [12]. Oilseed extraction techniques affect oil yield and quality [13,14]. Large-scale oil production from oil-bearing crops such as sunflower usually integrates both mechanical pressing and solvent extraction operations [15]. Mechanical screw pressing is the most used for oilseed pressing; however, its efficiency is low in terms of oil output, leaving a higher amount of the residual oil in the seed/press cake [16–18]. Several factors affect the mechanical screw press, including press geometry (screw and barrel), operating conditions (such as pressure) and seed pretreatments (such as temperature at the screw inlet, press cylinder and press head) [15,19–21]. The pressure developed in a screw press is rather difficult to control and predict [18]. In a uniaxial test by using a piston and a compression machine at a given pressure and speed, the oil-bearing material is put into a pressing vessel with holes at the bottom that allow the oil to escape while the press/seedcake is contained [22–24]. This process can be used to determine the required pressure/energy for obtaining the maximum oil yield from the bulk oil-bearing material and the residual oil in the press cake. The maximum oil yield is achieved at the first pressing, while the residual oil is gained through repetitive loading until no further pressing of the material is required (plastic deformation). The deformation energy is calculated from the area under the force–deformation curve according to the trapezoidal rule [22,25]. Compression factors (speed, force, vessel diameter and pressing height) and material pretreatments (heating temperature and heating time) thus influence oil recovery efficiency, residual oil in the press cake and the energy requirement [26,27]. Different physical and chemical parameters including moisture content, specific gravity, color, odor, acid value, viscosity, oxidative stability, triglyceride content, peroxide value, anisidine value, iodine value and free fatty acids, among others, are used to assess the compositional quality of vegetable oils [7,28,29]. These quality assessments are usually done with conventional techniques such as pycnometry, titration and standard AOAC methods [7,30]. Modern spectroscopic techniques including near-infrared (NIR), midinfrared (MIR), Fourier transform infrared (FTIR), Raman spectroscopy (RS), nuclear magnetic resonance (NMR) and hyperspectral imaging (HIS) are used to assess the quality of vegetable oils, but they are beyond the scope of this study [7,31]. This study, however, considered the titration/volumetric technique and standard AOAC methods to determine the chemical properties, namely, the acid value (AV), free fatty acids (FFAs) and peroxide value (PV) of the extracted oil samples. The AV measures the degree of oil spoilage in terms of FFAs from enzymatic activity [2,32]. The higher the AV, the higher the level of FFAs, which translates into decreased oil quality [33]. FFAs are the result of glycerin decomposition in oils [2]. High levels of FFAs cause rancidity as well as changes in the taste and color of the oil [34,35]. It is reported that during the oil refining process, FFAs are neutralized to reduce their undesirable effects/flavor [3,36]. Adeyanju et al. [34] and Cammerer and Kroh [37] published that high temperature, moisture content and presence of lipase are responsible for the formation of FFAs in fat-containing raw materials or oils. The PV measures the degree of either the occurrence of peroxidation or adulteration, which is used to evaluate the quality and stability of oils during storage [2,38,39]. A high peroxide value is an indicator of oxidation level, and the greater the peroxide value, the more oxidized the oil [40]. Oils with peroxide values higher than 9 meq O2/kg cause undesirable health problems by increasing reactive oxygen species as well as secondary products of lipid peroxidation that stimulate cardiovascular and inflammatory diseases [41,42]. Maximum levels of these chemical properties have been published in the Codex Alimentarius [36,43]. Generally, adequate information is still needed in a uniaxial process to understand the complexities associated with the mechanical screw pressing and for improving its efficiency as well as the quality of the oil output. Therefore, this study aimed to: determine the maximum oil yield from the bulk oilseeds and the residual oil content in the press cake through continuous loading, determine the physicochemical properties of the extracted oil samples using standard methods and describe the absorbance and transmittance spectra in the wavelength range of 325–600 nm using a UV–VIS spectrophotometer.
2. Materials and Methods
2.1. Samples and Determination of Moisture Content
Eight packets of cleaned hulled sunflower seeds (500 g each making a total of 4 kg)
were procured from a supermarket in Prague, Czech Republic. The samples were sealed
in two transparent plastic bags (Figure 1a) and kept at a laboratory temperature of 24 ◦C
and humidity of 25%. The initial moisture content of the samples was determined using
the conventional oven procedure at a temperature of 105 ◦C for 24 h [44–46]. The tests
were conducted twice, and the averaged moisture content value of 4.48 ± 0.19 (% w.b.)
was calculated [45]. The electronic balance Kern 440-35 (Kern & Sohn GmbH, Balingen,
Germany) with an accuracy of 0.01 g was used for weighing the samples.
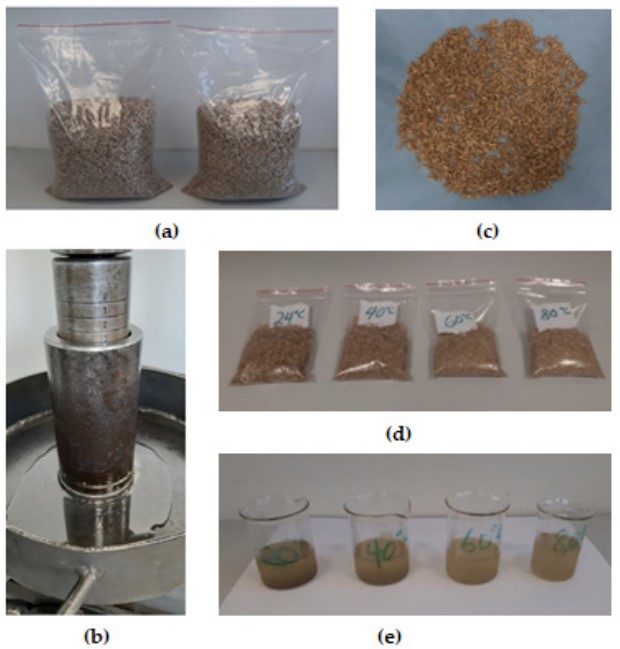
Figure 1. (a) Hulled sunflower seeds in sealed transparent plastic bags; (b) measured sample loaded in a pressing vessel with a plunger placed atop showing the extracted oil in a metal pan; (c) sample after first pressing spread out on a tissue; (d) samples after the fifth repeated pressing for each temperature to obtain the residual oil in the seedcake after the first pressing; (e) extracted oil in a beaker at each temperature of 24 ◦C (control), 40 ◦C, 60 ◦C and 80 ◦C.
2.2. Determination of Sample Oil Content The oil content of hulled sunflower seeds sample was determined by the Soxhlet extraction method [47–49]. Approximately 11 g of the sample weight was used. The tests were conducted twice, and the oil content value of 56.09 ± 2.67 (%) was obtained [45]. 2.3. Samples Pretreatment The oven (MEMMERT GmbH + Co. KG, Buechenbach, Germany) was used for the pretreatment of the samples at temperatures of 40, 60 and 80 ◦C before the compression tests to extract the oil. The temperature of 24 ◦C served as the control for the unheated sample. 2.4. Oil Extraction under Uniaxial Process Universal compression testing equipment (TEMPOS spol. s.r.o., Opava, Czech Republic (Machine Service), a ZDM 50, VEB Werkstoffprüfmaschinen Leipzig, Germany) and a pressing vessel of 60 mm diameter with a plunger were used to extract the oil from the hulled sunflower seed samples at a control temperature of 24 ◦C and heating temperatures of 40, 60 and 80 ◦C at a preset load of 40 kN (equivalent pressure of 14.147 MPa), speed of 4 mm/min and sample pressing height of 60 mm (sample volume of 16.965 × 10–5 m3 or an initial weight of 103.29 g to a final weight of 65.31 ± 0.70 g) (Figure 1b–e). The preset load (limit force) was determined from a preliminary test, being the curve with the serration effect, which was characterized by the ejection of the seedcake through the bottom holes of the pressing vessel (Figure 2). After the first pressing at 60 mm to obtain the maximum oil from the bulk seed samples, a repeated pressing was done to recover the residual oil in the seedcake until there was no need for further pressings (plastic deformation). The second, third, fourth and fifth repeated pressings were done at pressing heights of 50 mm, 40 mm, 35 mm and 30 mm, respectively. In all, at each temperature, five separate tests were conducted, making a total of 40 tests and repeated twice. From the compression tests and the data obtained, the oil yield, oil expression efficiency and deformation energy were calculated according to the following equations (Equations (1)–(3)) [25,50–52]:
𝑂𝑌𝐷=[(𝑚𝑂𝐿𝑚𝑆𝐷) 100]
(1) where OYD is the oil yield (%), and mOL is the mass of oil obtained as the difference of the mass of the seed cake and the initial mass of the sample mSD (g).
𝑂𝐸𝐹=[(𝑂𝑌𝐷𝑂𝐿𝐶) 100]
where OEF is the oil expression efficiency (%), and OLC is the percentage sample oil content (%) determined by the Soxhlet extraction method.
𝐸𝑁𝐺=∑𝑛=0𝑛=𝑖−1[(𝐹𝑛+1+𝐹𝑛2) (𝑥𝑛+1−𝑥𝑛)]
(3) where ENG is the deformation energy (J) characterized by the area under the force–deformation curve based on the trapezoidal rule, Fn+1 + Fn and xn+1 − xn are the compressive force (kN) and deformation (mm), n is the number of data points and i is the number of sections in which the axis deformation was divided. The deformation values were directly obtained from the compression data. The sample volume was calculated using Equation (4) [22]:
𝑉𝑆𝑃=[(π 𝐷24) 𝑃𝐻]
(4) where VSP is the sample volume (m3 ), D is the pressing vessel diameter (mm) and PH is the sample pressing height (mm).
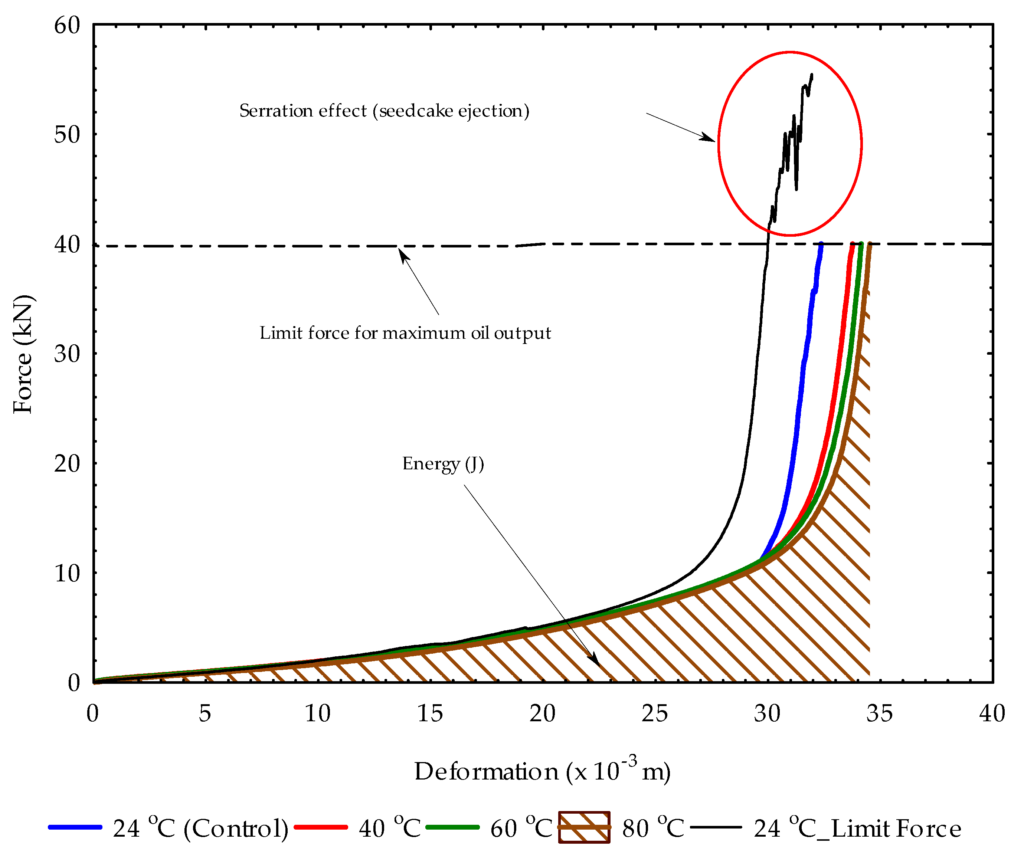
2.5. Determination of Oils Quality Parameters
2.6. Measurement of UV Spectral Parameters
2.7. Statistical Analyses
3. Results
3.1. Compression Curves at Initial and Repeated Pressings
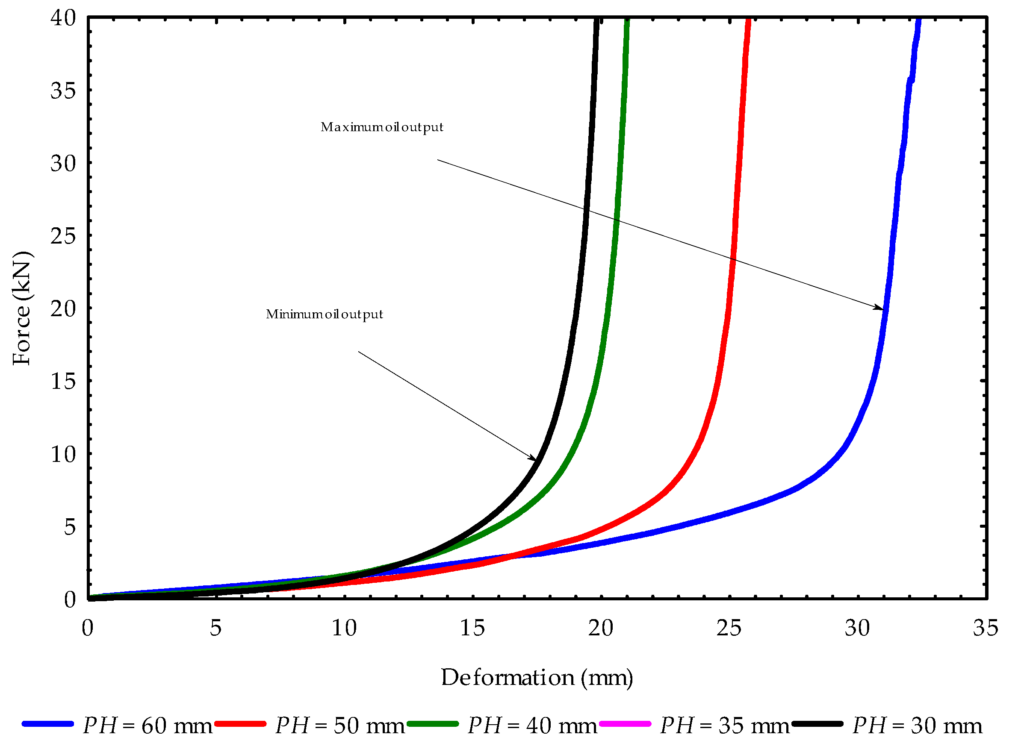
3.2. Calculated Parameters and Statistical Evaluation
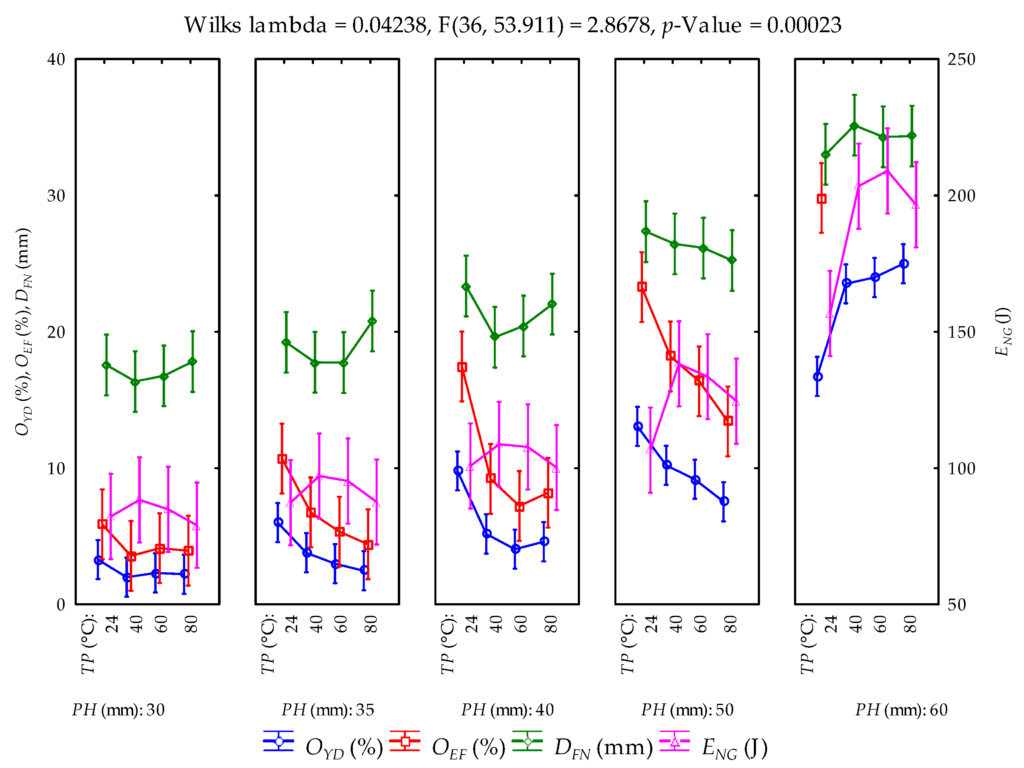
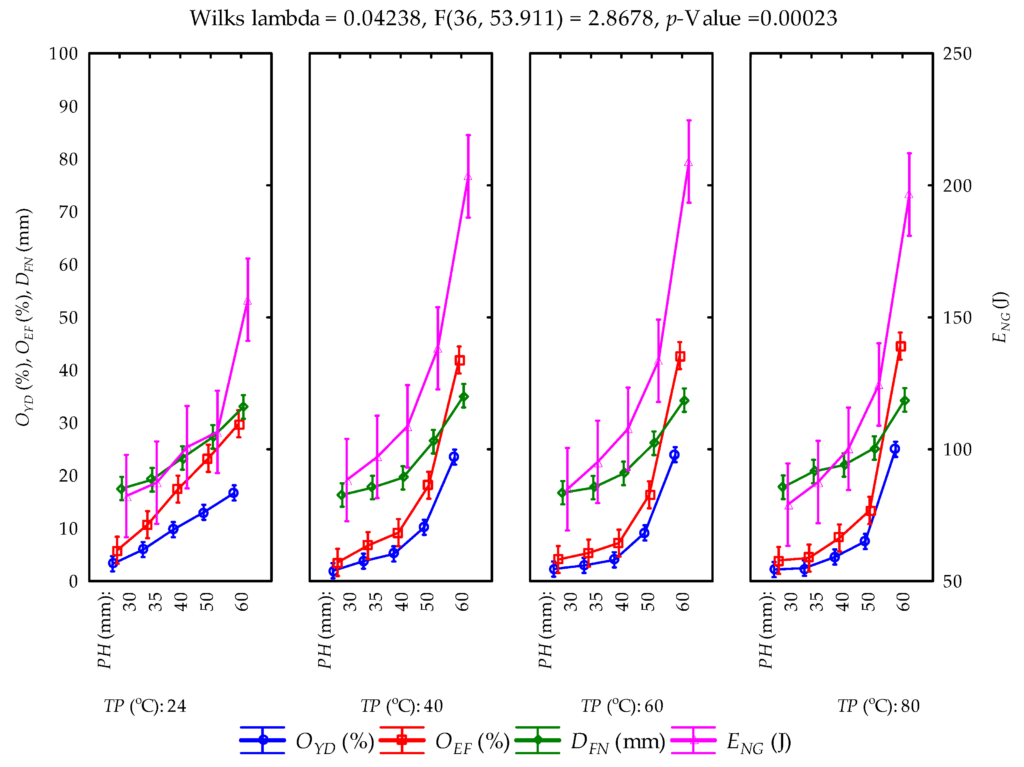
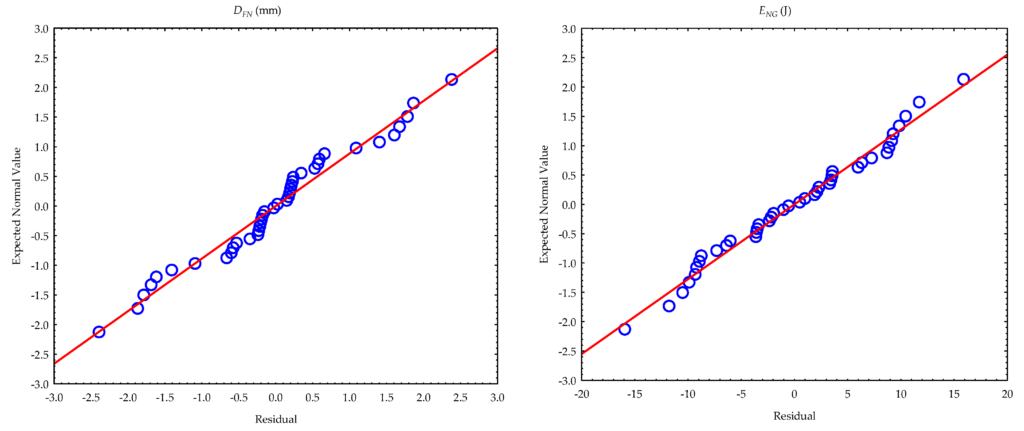
3.3. Quality Indicators of Oils and Statistical Evaluation
3.4. UV Spectral Properties of Oils
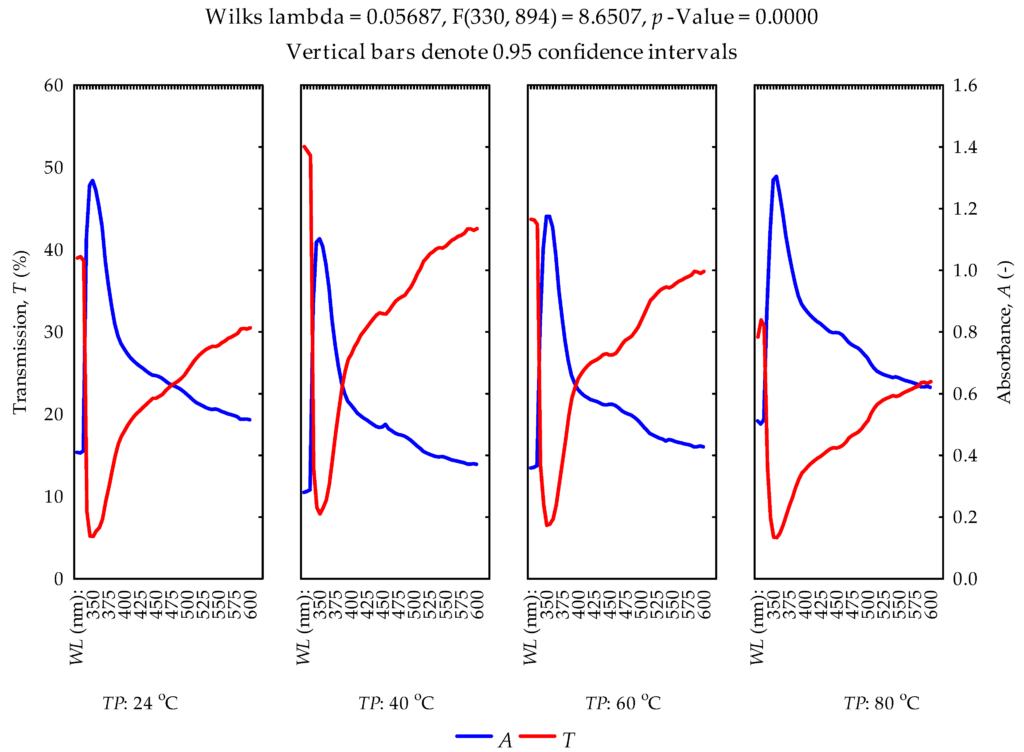
3.5. Supplementary Materials
4. Discussion
5. Conclusions
Supplementary Materials
Author Contributions
Funding
Institutional Review Board Statement
Informed Consent Statement
Data Availability Statement
Conflicts of Interest
References
- Stevenson, D.G.; Eller, F.J.; Wang, L.; Jane, J.L.; Inglett, G.E. Oil and Tocopherol content and composition of pumpkin seed oil in 12 cultivars. J. Agric. Food Chem. 2007, 55, 4005–4013. [Google Scholar] [CrossRef] [PubMed]
- Chatepa, L.E.C.; Uluko, H.; Masamba, K. Comparison of oil quality extracted from selected conventional and non conventional sources of vegetable oil from Malawi. Afr. J. Biotechnol. 2019, 18, 171–180. [Google Scholar]
- Gotor, A.A.; Rhazi, L. Effects of refining process on sunflower oil minor components: A review. OCL 2016, 23, D207. [Google Scholar] [CrossRef]
- Teixeira, M.R.; Nogueira, R.; Nunes, L.M. Quantitative assessment of the valorization of used cooking oils in 23 countries. Waste Manag. 2018, 78, 611–620. [Google Scholar] [CrossRef]
- Mitrea, L.; Teleky, B.-E.; Leopold, L.-F.; Nemes, S.-A.; Plamada, D.; Dulf, F.V.; Pop, I.-D.; Vodnar, D.C. The physicochemical properties of five vegetable oils exposed at high temperature for a short-time-interval. J. Food Compost. Anal. 2022, 106, 104305. [Google Scholar] [CrossRef]
- Ng, T.-T.; So, P.K.; Zheng, B.O.; Yao, Z.-P. Rapid screening of mixed edible oils and gutter oils by matrix-assisted laser desorption/ionization mass spectrometry. Anal. Chim. Acta 2015, 884, 70–76. [Google Scholar] [CrossRef]
- Rifna, E.J.; Pandiselvam, R.; Kothakota, A.; Rao, K.V.S.; Dwivedi, M.; Kumar, M.; Thirumdas, R.; Ramesh, S.V. Advanced process analytical tools for identification of adulterants in edible oils—A review. Food Chem. 2022, 369, 130898. [Google Scholar] [CrossRef]
- Dimitrijevic, A.; Horn, R. Sunflower hybrid breeding: From markers to genomic selection. Front. Plant Sci. 2018, 8, 2238. [Google Scholar] [CrossRef] [PubMed]
- Martinez, A.L.; Quiroz, F.J.; Carrera, A.D. Detection of Plasmopara halstedii in sunflower seeds: A case study using molecular testing. J. Saudi Soc. Agric. Sci. 2021, 20, 395–400. [Google Scholar]
- Dean, C. Coconut oil for Alzheimer’s. J. Am. Acad. Physician Assist. 2012, 25, 1–5. [Google Scholar]
- Sandupama, P.; Munasinghe, D.; Jayasinghe, M. Coconut oil as a therapeutic treatment for alzheimer’s disease: A review. J. Future Foods. 2022, 2, 41–52. [Google Scholar] [CrossRef]
- Kumar, K.A.; Viswanathan, K. Study of UV transmission through a few edible oils and chicken oil. J. Spectrosc. 2013, 2013, 540417. [Google Scholar]
- Liu, R.; Lu, M.; Zhang, Z.; Chang, M.; Wang, X. Evaluation of the antioxidant properties of micronutrients in different vegetable oils. Eur. J. Lipid Sci. Technol. 2020, 122, 1900079. [Google Scholar] [CrossRef]
- Cai, Z.; Li, K.; Lee, W.J.; Reaney, M.T.J.; Zhang, N.; Wang, Y. Recent progress in the thermal treatment of oilseeds and oil oxidative stability: A review. Fundam. Res. 2021, 6, 767–784. [Google Scholar] [CrossRef]
- Savoire, R. Screw pressing applications to oilseeds. Ref. Modul. Food Sci. 2017. [Google Scholar] [CrossRef]
- Savoire, R.; Lanoiselle, J.L.; Vorobiev, E. Mechanical continuous oil expression from oilseeds. A review. Food Bioprocess Technol. 2013, 6, 1–16. [Google Scholar] [CrossRef]
- Singh, K.K.; Wiesenborn, D.; Kangas, N.; Tostenson, K. Screw pressing characteristics of dehulled crambe seed. Trans. ASABE 2004, 47, 199–204. [Google Scholar] [CrossRef]
- Bogaert, L.; Mathieu, H.; Mhemdi, H.; Vorobiev, E. Characterization of oilseeds mechanical expression in an instrumented pilot screw press. Ind. Crops. Prod. 2018, 121, 106–113. [Google Scholar] [CrossRef]
- Mpagalile, J.J.; Clarke, B. Effect of processing parameters on coconut oil expression efficiencies. Int. J. Food Sci. Nutr. 2005, 56, 125–132. [Google Scholar] [CrossRef]
- Karaj, S.; Muller, J. Optimizing mechanical oil extraction of Jatropha curcas L. seeds with respect to press capacity, oil recovery and energy efficiency. Ind. Crops. Prod. 2011, 34, 1010–1016. [Google Scholar] [CrossRef]
- Romuli, S.; Karaj, S.; Latif, S.; Muller, J. Performance of mechanical co-extraction of Jatropha curcas L. kernels with rapeseed, maize or soybean with regard to oil recovery, press capacity and product quality. Ind. Crops. Prod. 2017, 104, 81–90. [Google Scholar] [CrossRef]
- Divisova, M.; Herak, D.; Kabutey, A.; Sigalingging, R.; Svatonova, T. Deformation curve characteristics of rapeseeds and sunflower seeds under compression loading. Sci. Agric. Bohem. 2014, 45, 180–186. [Google Scholar]
- Munson-Mcgee, S.H. D-optimal experimental designs for uniaxial expression. J. Food Process Eng. 2014, 37, 248–256. [Google Scholar] [CrossRef]
- Demirel, C.; Kabutey, A.; Herák, D.; Sedlaček, A.; Mizera, Č.; Dajbych, O. Using Box–Behnken Design Coupled with Response Surface Methodology for Optimizing Rapeseed Oil Expression Parameters under Heating and Freezing Conditions. Processes 2022, 10, 490. [Google Scholar] [CrossRef]
- Gupta, R.K.; Das, S.K. Fracture resistance of sunflower seed and kernel to compressive loading. J. Food Eng. 2000, 46, 1–8. [Google Scholar] [CrossRef]
- Kabutey, A.; Mizera, Č.; Dajbych, O.; Hrabě, P.; Herák, D.; Demirel, C. Modelling and Optimization of Processing Factors of Pumpkin Seeds Oil Extraction under Uniaxial Loading. Processes 2021, 9, 540. [Google Scholar] [CrossRef]
- Kabutey, A.; Herak, D.; Mizera, C.; Hrabe, P. Compressive loading experiment of non-roasted bulk oil palm kernels at varying pressing factors. Int. Agrophysics 2018, 32, 357–363. [Google Scholar] [CrossRef]
- Erum, Z.; Rehana, S.; Mehwish, A.H.; Anjum, Y. Study of Physicochemical Properties of Edible Oil and Evaluation of Frying Oil Quality by Fourier Transform-Infrared (FT-IR) Spectroscopy. Arab. J. Chem. 2014, 10, 3870–3876. [Google Scholar]
- Herchi, W.; Ammar, K.B.; Bouali, I.; Abdallah, I.B.; Guetet, A.; Boukhchina, S. Heating effects on physicochemical characteristics and antioxidant activity of flaxseed hull oil (Linum usitatissimum L.). Food Sci. Technol. 2016, 36, 97–102. [Google Scholar] [CrossRef]
- Tang, T.S. 8 Analysis of oleochemicals. Oleochem. Manu. Appl. 2001, 4, 227. [Google Scholar]
- de Souza, T.R.P.; Olenka, L.; Peternella, W.S. A study of degradation in vegetable oils by exposure to sunlight using Fourier Transform Infrared Spectroscopy. Mater. Sci. Appl. 2020, 11, 678–691. [Google Scholar] [CrossRef]
- Amadi, B.A.; Lele, K.C.; Duru, M.K.C. Extraction and characterization of vegetable oils from legume and palmae; using African Oil Bean (Pentaclethra macrophylla) and ‘Akwu Ojukwu’ (Elais guineensis) respectively. Am. J. Biol. Life Sci. 2013, 1, 7–10. [Google Scholar]
- Katkade, M.B.; Syed, H.M.; Andhale, R.R.; Farooqui, A.S. Comparative study of physicochemical properties of different edible vegetable oils. J. Sci. Agric. Eng. 2018, 8, 235–238. [Google Scholar]
- Adeyanju, J.A.; Ogunlakin, G.O.; Adekunle, A.A.; Alawode, G.E.; Majekolagbe, O.S. Optimization of oil extraction from coconut using response surface methodology. J. Chem. Pharm. Res. 2016, 8, 374–381. [Google Scholar]
- Ifa, L.; Syarif, T.; Sartia, S.; Juliani, J.; Nurdjannah, N.; Kusuma, H.S. Techno-economics of coconut coir bioadsorbent utilization on free fatty acid level reduction in crude palm oil. Heliyon 2022, 8, e09146. [Google Scholar] [CrossRef]
- Di Pietro, M.E.; Mannu, A.; Mele, A. NMR determination of free fatty acids in vegetable oils. Processes 2020, 8, 410. [Google Scholar] [CrossRef]
- Cammerer, B.; Kroh, L.W. Shelf life of linseeds and peanuts in relation to roasting. Food Sci. Technol. 2009, 42, 545–549. [Google Scholar] [CrossRef]
- Adejumo, B.A.; Inaede, S.G.; Adamu, T.S. Effect of moisture content on the yield and characteristics of oil from valiculatum and V. calycinium. Pac. Sci. 2013, 48, 458–463. [Google Scholar]
- Okene, E.O.; Evbuomwan, B.O. Solvent extraction and characteristics of oil from coconut seeds using alternative solvents. Int. J. Eng. Technol. Res. 2014, 2, 135–138. [Google Scholar]
- Atinafu, D.G.; Bedemo, B. Estimation of total free fatty acid and cholesterol content in some commercial edible oils in Ethiopia, Bahir DAR. J. Cereals Oilseeds 2011, 2, 71–76. [Google Scholar] [CrossRef]
- Lobo, V.; Patil, A.; Phatak, A.; Chandra, N. Free radicals, antioxidants and functional foods: Impact on human health. Pharmacogn. Rev. 2010, 4, 118–126. [Google Scholar] [CrossRef] [PubMed]
- Konuskan, D.B.; Arslan, M.; Oksuz, A. Physicochemical properties of cold pressed sunflower, peanut, rapeseed, mustard and olive oils grown in the Eastern Mediterranean region. Saudi J. Biol. Sci. 2019, 26, 340–344. [Google Scholar] [CrossRef] [PubMed]
- Codex Alimentarius Commission. Fats, Oils and Related Products, 2nd ed.; Food and Agriculture Organizaation of the United Nations: Rome, Italy, 2001; Volume 8. [Google Scholar]
- ISI. Indian Standard Methods for Analysis of Oilseeds. In Indian Standard Institute; IS: 3579; ISI: New Delhi, India, 1966. [Google Scholar]
- Blahovec, J. Agromatereials Study Guide; Czech University of Life Sciences Prague: Prague, Czech Republic, 2008. [Google Scholar]
- Keneni, Y.G.; Hvoslef-Eide, A.K.T.; Marchetti, J.M. Mathematical modelling of the drying kinetics of Jatropha curcas L. seed. Ind. Crops. Prod. 2019, 132, 12–20. [Google Scholar] [CrossRef]
- Niu, L.; Li, J.; Chen, M.S.; Xu, Z.F. Determination of oil contents in Sacha inchi (Plukenetia volubilis) seeds at different developmental stages by two methods: Soxhlet extraction and time-domain nuclear magnetic resonance. Ind. Crops. Prod. 2014, 56, 187–190. [Google Scholar] [CrossRef]
- Mohammadpour, H.; Sadrameli, S.M.; Eslami, F.; Asoodeh, A. Optimization of ultrasound-assisted extraction of Moringa peregrina oil with response surface methodology and comparison with Soxhlet method. Ind. Crops. Prod. 2019, 131, 106–116. [Google Scholar] [CrossRef]
- Gürdil, A.K.G.; Kabutey, A.; Selvi, K.C.; Hrabe, P.; Herak, D.; Frankova, A. Investigation of heating and freezing pretreatments of mechanical, chemical and spectral properties of bulk sunflower seeds and oil. Processes 2020, 8, 411. [Google Scholar] [CrossRef]
- Deli, S.; Farah Masturah, M.; Tajul Aris, Y.; Wan Nadiah, W.A. The effects of physical parameters of the screw press oil expeller on oil yield from Nigella sativa L. seeds. Int. Food Res. J. 2011, 18, 1367–1373. [Google Scholar]
- Chanioti, S.; Constantina, T. Optimization of ultrasound-assisted extraction of oil from olive pomace using response surface technology: Oil recovery, unsaponifiable matter, total phenol content and antioxidant activity. LWT Food Sci. Technol. 2017, 79, 178–189. [Google Scholar] [CrossRef]
- Lysiak, G. Fracture toughness of pea: Weibull analysis. J. Food Eng. 2007, 83, 436–443. [Google Scholar] [CrossRef]
- Demirel, C.; Kabutey, A.; Herák, D.; Hrabě, P.; Mizera, Č.; Dajbych, O. Optimizing Uniaxial Oil Extraction of Bulk Rapeseeds: Spectrophotometric and Chemical Analyses of the Extracted Oil under Pretreatment Temperatures and Heating Intervals. Processes 2021, 9, 1755. [Google Scholar] [CrossRef]
- Orozco, F.D.A.; Sousa, A.C.; Araujo, M.C.U.; Domini, C.E. A new flow UV-Vis kinetics spectrophotometric method based on photodegradative reaction for determining the oxidative stability of biodiesel. Fuel 2020, 26, 116–197. [Google Scholar]
- Statsoft Inc. STATISTICA for Windows; Statsoft Inc.: Tulsa, OK, USA, 2013. [Google Scholar]
- Khan, L.M.; Hanna, M.A. Expression of oil from oilseeds—A Review. J. Agric. Eng. Res. 1983, 28, 495–503. [Google Scholar] [CrossRef]
- Li, W.G.; Sun, X.L.; Zu, Y.G.; Zhao, X.H. Optimization peony seed oil extraction process at suitable temperature and physicochemical property analysis. Bull. Botan. Res. 2020, 40, 73–78. [Google Scholar]
- Deng, R.; Gao, J.; Yi, J.; Liu, P. Could peony seeds oil become a high-quality edible vegetable oils? The nutritional and phytochemistry profiles, extraction, health benefits, safety and value-added-products. Food Res. Int. 2022, 156, 111200. [Google Scholar] [CrossRef] [PubMed]
- Dodoo, D.; Adjei, F.; Tulashie, S.K.; Adukpoh, K.E.; Agbolegbe, R.E.; Gawou, G.; Manu, G.P. Quality evaluation of different repeatedly heated vegetable oils for deep-frying of yam fries. Meas. Food. 2022, 7, 100035. [Google Scholar] [CrossRef]
- Hoffmann, G. The Chemistry and Technology of Edible Oils and Fats and Their High-Fat Products; Academic Press: New York, NY, USA, 1989; pp. 63–68. [Google Scholar]
- Reuber, M. New Technologies for processing Crambe abyssinica. M.S. Thesis, Iowa State University, Ames, IA, USA, 1992. [Google Scholar]
- Singh, K.K.; Wiesenborn, D.P.; Tostenson, K.; Kangas, N. Influence of moisture content and cooking on screw pressing of crambe seed. JAOCS 2002, 79, 165–170. [Google Scholar] [CrossRef]
- Choo, W.S.; Birch, E.J.; Dufour, J.P. Physicochemical and stability characteristics of flaxseed oils during pan-heating. JAOCS 2007, 84, 735–740. [Google Scholar] [CrossRef]
- Ajai, A.I.; Adedokun, T.A.; Inobeme, A.; Jacob, J.O. Determination of physicochemical properties of selected vegetable oils in Minna. FUW Trends Sci. Technol. J. 2016, 1, 475–478. [Google Scholar]
- Vicentini-Polette, C.M.; Ramos, P.R.; Goncalves, C.B.; De Oliveira, A.L. Determination of free fatty acids in crude vegetable oil samples obtained by high-pressure processes. Food Chem. X 2021, 12, 100166. [Google Scholar] [CrossRef]
- Eke-Ejiofor, J.; Beleya, E.A.; Allen, J.E. Effect of variety on the quality parameters of crude soybean oil. Am. J. Food Sci. Technol. 2021, 9, 69–75. [Google Scholar]
- Nduka, J.K.C.; Omozuwa, P.O.; Imanah, O.E. Effect of heating on the physiological properties of selected vegetable oils. Arab. J. Chem. 2021, 14, 103063. [Google Scholar] [CrossRef]
- Tiffour, I.; Dehbi, A.; Mourad, A.-H.I.; Belfedal, A. Synthesis and characterization of a new organic semiconductor material. Mater. Chem. Phys. 2016, 178, 49–56. [Google Scholar] [CrossRef]
- Gonzalez, M.F.; Barbero, G.F.; Alvarez, J.A.; Ruiz, A.; Palma, M.; Ayuso, J. Authentication of virgin olive oil by a novel curve resolution approach combined with visible spectroscopy. Food Chem. 2007, 220, 331–336. [Google Scholar] [CrossRef] [PubMed]
- Wang, H.; Xin, Y.; Wan, X. Spectral detection technology of vegetable oil: Spectral analysis of porphyrins and terpenoids. Spectrochim. Acta Part A Mol. Biomol. Spectrosc. 2021, 261, 119965. [Google Scholar] [CrossRef]
- Altman, D.G.; Bland, J.M. Standard deviations and standard errors. BJM 2005, 331, 903. [Google Scholar] [CrossRef][Green Version]